TL;DR: In summary, fossil fuels, as finite resources, will peak and decline in the next decades. However, they are extremely difficult to replace, so it’s unlikely that alternatives can replace them at the scale required. You can check the short version of the post.
This is part 1 of 3 different EA forum posts talking about energy depletion as an important topic that will shape the future:
1 - Decline of fossil fuels and why alternative energy sources will probably not scale up
2 - Consequences: The role of investment, impact on economic growth and systemic risks. Plus, what that means for EA causes.
3 - What we can do, what we can't do, and why few people really anticipate this problem
Note: The original post was too long, so I divided it in 3 to avoid that (energy limits, consequences, what to do). The scope here is very broad, so the posts will only contain the overall reasoning and main arguments. If you are doubtful about a specific point, I wrote detailed sections that I added to an Additional Document (it's 140 pages, so I think I have covered the main counterarguments here). You can engage in comments in this Doc. For instance, you can read “Have other EAs addressed this topic?” in this section.
A quick introduction on energy
It’s often hard to see the importance of energy, so here’s a quick intro on it. Energy, simply put, defines the capacity to do work in a system (see here for more details). We need it to do basically anything. As Richard Heinberg puts it in his book Power: "if you want to understand any ecosystem or human society, a good rule of thumb is to follow the energy." Indeed, what all life forms are doing is to capture energy from their surroundings (in the form of sunlight or food) and to dissipate it to maintain steady conditions. Beings that are better at getting access to energy have more options, better survival and more “wealth” - however wealth manifests for that species. The most important events in human history came from the use of a new source of energy: first with the use of fire, second with agriculture (that allowed us to gather more energy from plants and livestock), and then, for the industrial revolution, the use of fossil fuels — coal, oil and gas. The big difference is that the latter is finite.
One of the things that determines the usefulness of an energy is its density. Most of the energy on Earth comes from the sun, but it exists in different shapes. Energy is not “produced”, it is collected from more or less dense sources. Wood, which stored energy from the sun for decades through photosynthesis, is useful because it is compact (easy to collect and put into a stove). On the other hand, heat scattered in the atmosphere is much harder to use (imagine how valuable a kilogram of flour is if it’s scattered over an entire field).
What makes fossil fuels really valuable — and hard to substitute — is that they are extremely concentrated forms of solar energy. You can see them as tons of plants compressed and cooked under the ground over millions of years - finding them was like accessing a huge battery. A single barrel of oil contains the equivalent of 5 years of human work, after conversion losses. In “Energy and Civilization: A History” (p.421), Vaclav Smil details how “countries with a relatively easy access to fuels that could be produced and distributed with less energy than the previous dominant source enjoyed faster economic growth, with its welcome correlates of greater prosperity and higher quality of life“. This was the case of the Dutch Republic’s Golden Age with peat, during the XVIIth century; the British empire with coal and coke; and the USA after 1870, fueled by abundant petroleum and coal.
The role of fossil fuels in making industrial civilization
These fossil fuels allowed for everything the industrial civilization provided, good or bad: synthetic fertilizers, modern hospitals and easy transportation, but also environmental destruction, abundant explosives for the two world wars, or factory farming. We forgot the origin of all of this: abundant, cheap and dense energy. It’s uncertain that the modern industrial civilization could have started (or restarted) without fossil fuels. Today, the amount of energy used by, say, the average French, represents the equivalent of 400 slaves working full time. For an American, this is about twice as much.
Today, despite decades of activists calling for an energy transition, the main sources in use are still oil, coal and gas, at about 85%. Solar and wind, which are less dense and more difficult to collect, store and transport, represent only 1.36% of primary energy in 2019.
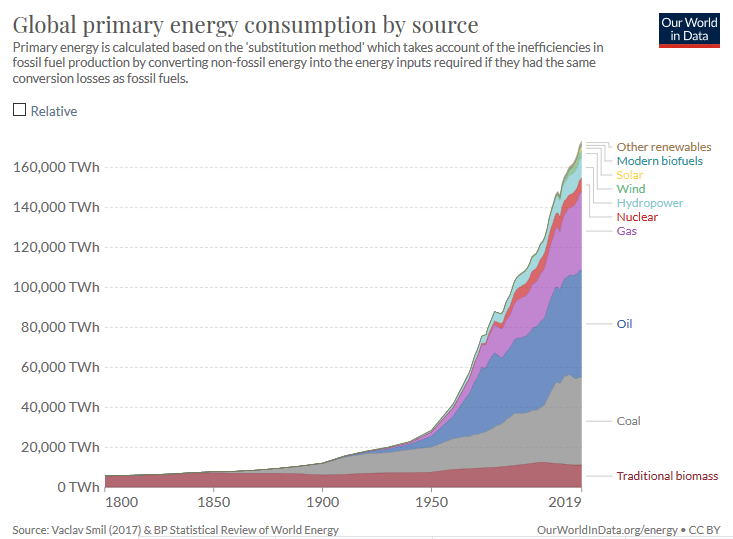
I think it is important to understand why getting out of fossil fuels is really hard. Energy is rather invisible in our daily lives, so please allow us to present an example. Let’s take cornflakes: how would you produce them without fossil fuels?
“The story of a bowl of cornflakes begins on a farm—say, in Iowa—which grew corn using diesel fuel (derived from oil) to power machinery for plowing, seeding, harvesting, and production. Oil-powered machines were also used for applying nitrogen fertilizer and herbicides, and even earlier for mining, transporting, and processing phosphorus and potassium fertilizers. After harvest, the corn was trucked (using oil) to a grain elevator, where it was dried (natural gas); then it was transported by rail (oil) to Michigan for processing. After processing, the cornflakes were packaged in a plastic bag (oil) and cardboard boxes (wood). The boxes of cornflakes were trucked (oil) to a warehouse (most operations including lighting, cooling, and pallet trucks are electric), then to the store and transported home—most likely by car (oil). [...] The single largest energy input is in the form of fertilizer, which relies on a process that transforms fossil fuels (usually natural gas) into ammonia.”
The source is here. We can technically replace these elements, but at what scale? Will it be fast enough? And who will be able to afford it?
To get the wider picture, one of the best videos I’ve ever seen is this extraordinary 30 minutes movie called “The Great Simplification” by Nate Hagens. As he points out:
“Most people believe that money is real wealth, yet everything we spend money on requires energy to mine, create, deliver, run, maintain, and dispose of. In this way, money is ultimately a direct claim on energy and resources. Our economic stories assert that with more money we can create more of anything. The truth is, we cannot create energy. We both extract it and burn it faster by using technology and printing money. Natural capital, particularly energy, is the true foundation of our monetary systems. As we create more money, we don't create more resources,we merely access them faster.”
Can this go on forever? At the current growth rate, this would mean using as much energy in the next 30 years as we used over the last 10 000 years. This sounds daunting. We will see that we are starting to hit limits, and that we are not necessarily prepared to face the changes this implies.
My methods and background
Feel free to skip that part and get to “Peak oil” if you just want to get to the heart of the topic.
My background
I do not come from an initial energy-related background, so my initial assumption on the topic was fairly basic: that energy depletion was not a main issue, and that we had to transition toward renewables for the climate. However, in France, many experts are working on that topic, such as Jean-Marc Jancovici, Gael Giraud and especially Arthur Keller. I was lucky enough to hear what they had to say, and I started digging more into energy depletion. Thus, I am not doing any original calculations here: I am mostly aggregating what many experts on the topic have been saying. I have been advised to write this post by French EA folks interested in this, and I spent more than a year working on this post, sinking hundreds of hours in order to get as broad of a picture as I could (and it turned out the current energy crisis started in the meantime). My worldview evolved a lot during the writing, but so far what these French energy experts pointed out seems fairly solid.
My approach - aim of the post
This post does not aim to provide a complete overview of the advantages and drawbacks of all energy sources, which would be very, very long. These reviews are important, but, in my opinion, not very engaging for the reader. I’ll then focus on limits here. I also do not have the skills to produce precise scenarios with error bars, or to make complicated modelizations and calculations, like cost-effectiveness estimates. My aim here is more “here’s why I care about this topic, and the strongest arguments in the literature that support this position” (steelmanning).
One of the reasons I got very different results than most energy transition scenarios is that I try to have as large of a “big-picture” as I can, reading on a lot of topics, and making connections (and being lucky that there are people in France warning about that). This is not the case of most of the literature, which has a very narrow focus (“how many solar panels and nuclear plants do we need to replace oil?”). I often find limits not in invalid data, but rather because another related topic has been neglected. For instance, most energy transition models do not even allow for an “energy descent scenario”, as they do not include mineral depletion, or the relationship between the economy, energy and investment (as we’ll see).
Limits of my approach
I prefer reading people who already synthesized things, but this means I have less depth about purely technical topics. I also tackle many complicated topics at once, so it’s easy to make mistakes. It’s often very hard to get good data here (see also Post 3, “Why is this neglected?”), so there are probably many things here that are incomplete, or even false. As I learned more, I even did some “back-and-forth” on several issues - like on mineral depletion, I went from “it’s important” to “no big matter” to “it IS a big deal”. This is a normal part of the process. To counter that, I tried to ask many people to review what I wrote. I really want to thank Yvan Denis for his help, the post would be of much lower quality without his reviews. The input of ALLFED co-founder Dave Denkenberger, an EA energy expert (and optimistic about the topic), has also been invaluable in this process (although we still don’t agree on several points).
Moreover, the hard part here is that most of the problems I mention aren’t purely technical - a solution might theoretically work, but that doesn’t mean that it will be used at scale. This means that the implicit hypotheses we all have about how society works matter a great deal (I’ll detail mine at the end). If our assumption is that markets will always adapt and technological innovation will always save the day, then energy depletion is a scenario we have a hard time to even consider.
As Nate Hagens puts it: “There are things that can happen, things that can’t happen, and things that won’t happen. Telling the first from the last is the tricky part.”
Peak oil - When will oil start declining ?
Peak oil refers here to the moment where oil production reaches its maximum and then declines. As oil is a finite resource, it will inevitably decline at some point - the issue is finding out when. See this podcast for a more complete introduction on oil. There have been many instances where a peak was announced, but many of them were too early: in the past, new oil discoveries or additional technology improvements managed to keep the specter of peak oil at bay.
However, the size of newly discovered and developed fields tends to decrease over time: conventional discoveries have fallen to their lowest levels in 70 years, and we extract about 3 times more oil than we discover, despite comparatively higher prices and better technology. This is unsustainable. The reason for that is that oil extraction proceeds according to the low-hanging-fruit principle: the highest-quality and easiest-to-get resources are usually harvested first. That means that the remaining oil fields are of lowest quality, in extreme locations (like in the Arctic), in smaller quantities, offshore, or costly to extract (like shale oil). Up to this day, improvements in technology have managed to compensate, but we are hitting limits.
According to the International Energy Agency (IEA), the peak of conventional oil was reached in 2008 (the oil found in oil wells). Most countries have seen their oil production decline, and most of the growth in the last 10 years was due to unconventional oil, mostly US shale oil. The issue with shale oil is that it is much more costly (the industry lost $280 billion since 2007), less energy efficient, and depleting faster (wells production decline by 90% after 3 years). It also took a big blow with the pandemic. In 2020, nearly two-thirds of U.S. energy company executives polled by the Federal Reserve Bank of Dallas believed that U.S. crude oil production had peaked. Russia also announced it peaked in 2019. Even Saudi Arabia announced that it would reach its peak in 2027. This is a sign that the “easy oil” has run out.
As a consequence, a report for the French Ministry of the Army came to the following conclusion: “For lack of reserves large enough to make up for the current production decline, total oil supplies to the European Union are likely to drop by 10 to 20% over the 2030 decade, as compared to its current 2019 level.” Even a high tight oil production would not eliminate such a hazard. Oil Major Total is warning that the world could find itself with a shortfall of supply of 10 million barrels per day between now and 2025 (about a tenth of global production), due to continued underinvestment in the industry, the OPEC+ pact, and cracks in the U.S. shale business model. The maximum in oil production, so far, has been reached in November 2018 - a whole 15 months before Covid destroyed oil demand. It’s even possible that we might never reach this level again.
Net energy returns are declining
An additional trend is that we need more and more energy to extract the remaining oil supplies. Today, 15% of the energy is used to extract more energy. The following paper estimates that in 2050, this should be closer to 50%, as seen in yellow on the graph below:
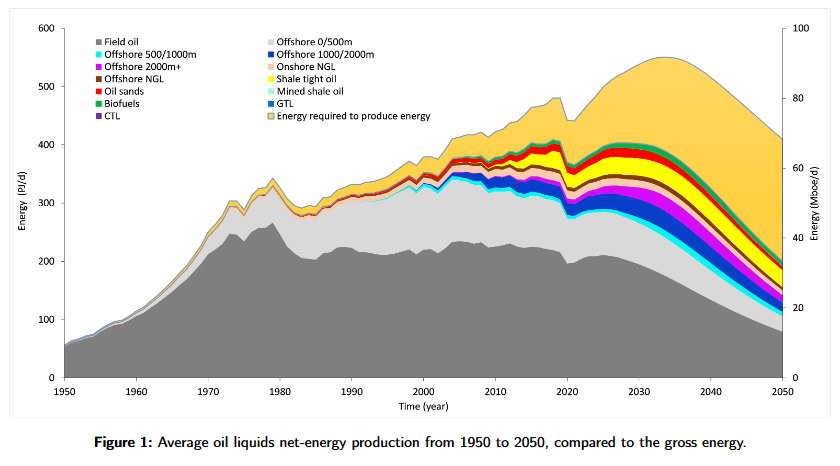
The report estimates that oil production will be on a plateau until 2030 and then decline. Since the orange parts cannot be used to run society, but only to maintain production, the authors call this “energy cannibalism”. There is a feedback loop going on here. Investment will probably go down as well, given that profits will take longer and longer to pay off (we’ll see in the next post that even if prices go up, that won’t be enough to compensate). This is also the case for natural gas, which should reach its peak around 2035 according to this paper: the amount of energy used to extract energy should grow from 6% to 24% in 2050.
For coal, figures are older and harder to come by, so I must admit I have more uncertainties. Figures of 250 years left are overblown, given recent reevaluations, but I don’t know how close the unavoidable decline is. When taking into account coal’s energy content, we still haven’t topped 2013 values - but some technological improvements are still possible. Some announce a peak in China’s production, the biggest producer, by 2030. There is more coal left than oil, though, which means it will last longer, but it is getting less and less affordable.
A peak does not mean that the resource will suddenly “run out”, but that it won’t be accessible at the scale required — only a smaller and smaller fraction of the population will be able to afford it. All of this means that at some point in the near future, we will get less and less energy from fossil fuels every year, not more. However, I have more uncertainty as for the exact date (this depends on what happens after Covid), and the rate of decline after that.
You can find here the complete “When can we expect a peak of fossil fuels?” section with all the links and details (and more data on coal and gas).
The current energy crisis
An additional note on the current situation: You may have noticed that we’re facing the biggest energy crisis in many decades, with supply chains seizing up and products made from or with oil and gas (notably fertilizers) suddenly becoming scarce and expensive. The International Energy Agency (IEA) forecasts that the ongoing war and the U.S. sanctions may together reduce Russian oil exports by at least 3 million barrels per day—more than 4% of global supplies, which is a huge chunk of the delicately balanced world energy market. Some energy analysts are forecasting that oil prices could spike up to $200 per barrel later this year, exacerbating inflation and triggering a global recession. The energy crisis today is “comparable in intensity, in brutality, to the oil shock of 1973,” France’s Economy and Finance Minister Bruno Le Maire said. French president Macron said that this was the "end of abundance". There are more details about that in this section.
Can renewable energy save the day?
The growth rate of renewables has been impressive, especially because costs dropped substantially as technology improved - annual growth rates since 2000 have been 52% for solar, 24% for wind. Extrapolation of past trends seems to indicate that renewables will be more abundant and cheaper than fossil fuels. (One should note that the same thing doesn’t apply to nuclear or carbon capture, whose costs didn't change much in the past). So if the current growth rate of renewables can be sustained for a long time, then the issue would be solved.
However, suppose that we were to use such a method in the 1950s. We would have concluded that oil was going to last forever with prices staying extremely low. This is not what happened. This method couldn't have predicted the 1970s oil shocks (and the associated financial crisis), or the 2008 financial crisis (where oil prices went from 20$ to 140$ between the years 2002 to 2008, see more details here). So the question is: where are the limits?
The Geological Survey of Finland (GTK) has done the most complete overview of the topic that I’ve ever managed to find. They checked what would be needed to replace fossil fuels by 2050 (you can read here the summary / longer summary / full article). They found that this amount of power stations were required:
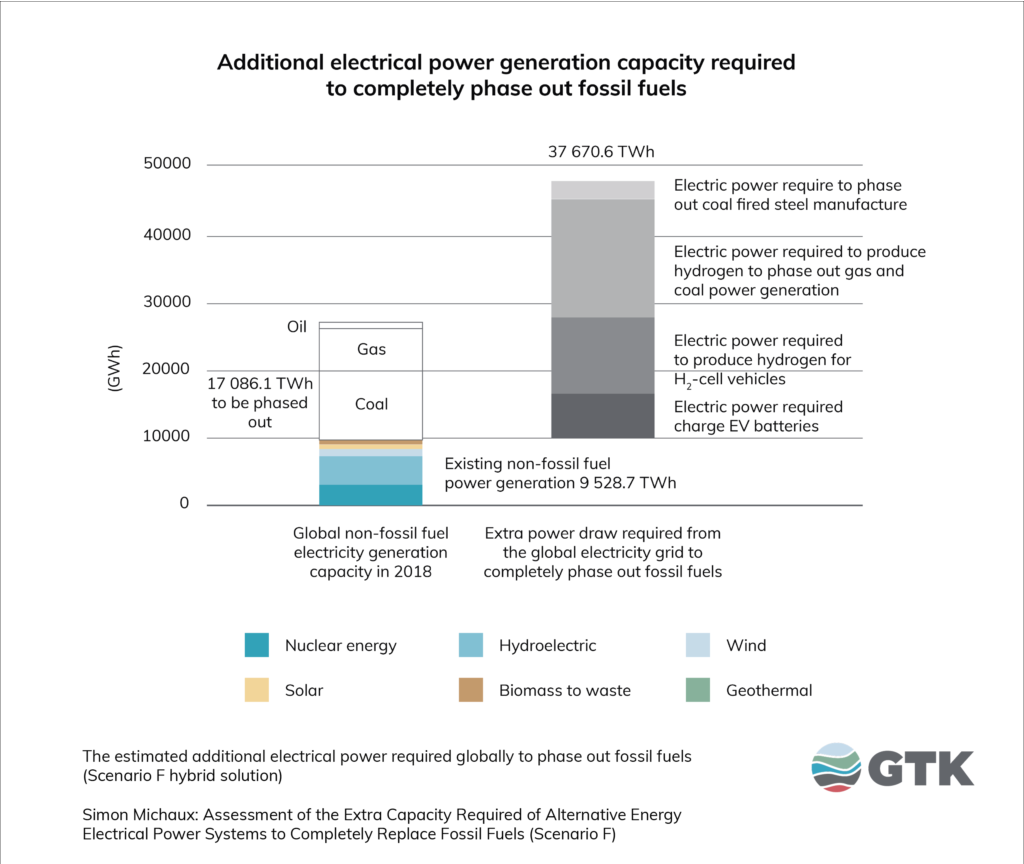
This means that an extra 221 594 new power plants will be needed to be constructed and commissioned. To put this in context, the total power plant fleet in 2018 (including fossil fuel plants) was only 46 423 stations (you need 213 average sized solar PV array farms to replace an average coal fired power station). Here’s their conclusion:
“Many of the solutions discussed in the open literature might work quite well at a comparatively small-scale but cannot function when scaled-up to a global scope to mimic the size of the existing fossil fuel sourced system. Usually, the bottleneck making this happen is the quantity of minerals required, the manufacturing capacity, or simply the required time to roll out production”. Moreover, “most analysts examine only one part of the ecosystem or only one function in isolation, where what is really required is a holistic systems network engineering approach that honors the inherent complexity”.
Let’s check that point by point.
Note: The following section assumes that investment will not increase considerably, and does not tackle adaptation (for instance, if we were to voluntarily use less cars). We’ll detail that in the next post (or you can check the detailed section here). We’ll also address substitution in the next post.
Minerals are finite too
First, the energy transition needs a lot of metals and materials to take place (for batteries, photovoltaic cells, electronic components, and so on). With the world renewables, the concept of infinity pops to mind - if we could harvest all of the sun’s energy, there would be no issue here. But the difficult part here is to actually harvest this energy, and build the items that do this. Today’s renewables, especially wind and solar, are complex objects that rely on long supply chains and many materials - a wind turbine relies on 8000 different parts. You also need metals to extend and reinforce the electric grid to handle intermittency, and to connect to the places with the most sun and wind resources.
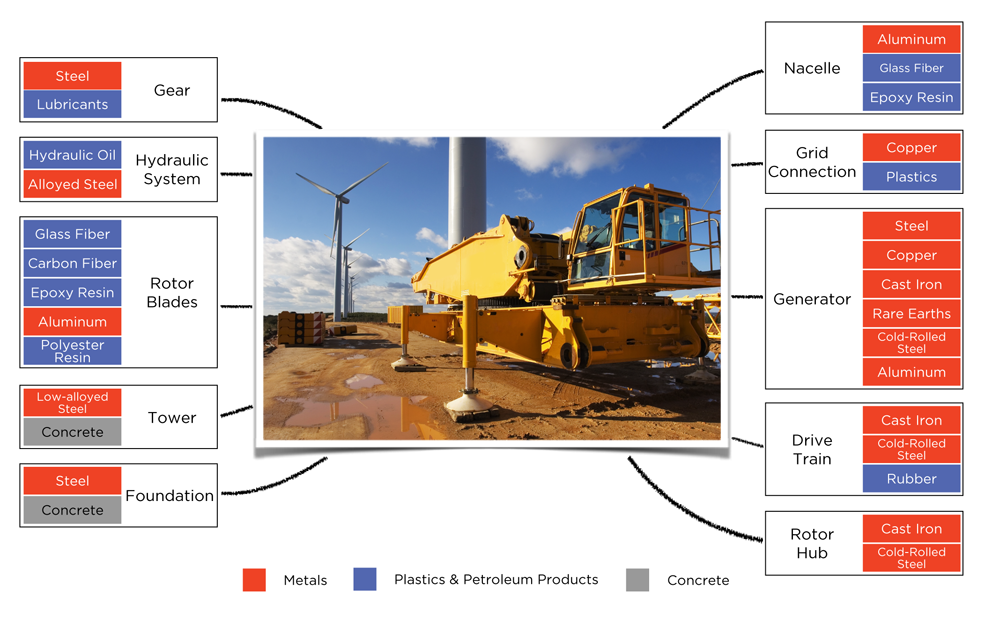
Materials required in the manufacturing and operation of a wind turbine (source)
Hence, a more accurate option would be calling them ‘rebuildables' instead. Solar panels and wind turbines have a lifespan of 25-30 years, and batteries have a lifespan of 8-10 years. So even if we managed to generate an entire generation of these devices, we’d have to do the same thing every 30 years.
It’s possible to recycle some of these metals to ease the issue, but recycling has limits. It cannot be 100% efficient, as the output is of lower quality at every iteration (hence the best recycling rates are rarely above 60-70%). Metals in high-tech products have lower recycling rates (<1%) because they are dispersed (used in alloys or in small quantities), so solving the issue with more complex technology often means losing more materials. For more, see the detailed section on recycling.
An energy transition, then, would imply the mining of a lot of metals. There are several limits here:
- Energy limits: As Ugo Bardi shows in the book Extracted: “the limits to mineral extraction are not limits of quantity; they are limits of energy.” Extracting minerals takes energy, for mining trucks (diesel, hard to electrify), grinding, refining and smelting (mostly gas and coal). Ore grade has been going down in the last decades, and the more dispersed the minerals are, the more energy is needed. As Michaux 2021 puts it, “rates of mining will peak and decline as a function of available energy, not as a function of available mineral resources”.
- Short-term limits: According to the IEA, a reference in the domain, “today’s mineral supply and investment plans fall short of what is needed to transform the energy sector, raising the risk of delayed or more expensive energy transitions”. They also point out that the mining industry cannot expand itself in a hurry (it takes on average 16.5 years to move mining projects from discovery to first production).
- Medium-term: If we were to replace one generation of electric cars and do seasonal storage of intermittent electricity with lithium batteries, we would need much more than what mineral reserves can provide (see the GTK report above). This solution “will not work” (sic), but this is a problem as it was the main plan put forward by governments and international agencies. “It is not clear how this power buffer could be delivered with an alternative system”. There are solutions like pumped hydro and compressed air storage (CAES) but they have trouble scaling up (more on storage here).
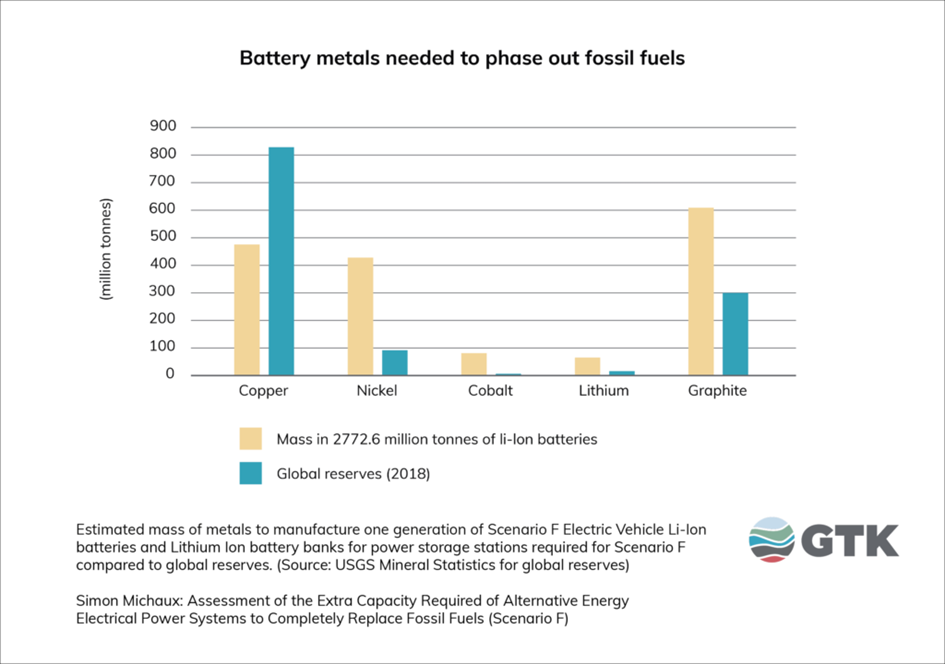
- Long-term: Over the long term, most minerals are expected to peak within the century. Only 9 of them (and, ironically, rare earths) have resources for more than 100 years, plus iron and aluminium. It’s possible that more reserves are discovered, but even doubling them would mean a 20 years delay with an exponential consumption. If almost all metals are facing some tension at some point, then substituting one to another only delays the problem.
All of this is detailed in the “Are there enough metals available for a transition toward 100% renewables?” section.
Hydroelectricity, however, is a good option, and relies less on materials (although it emits significant greenhouse gasses in tropical zones and disrupts the flow of rivers). However, they have a limited growth potential, constrained by geography: most of the good spots for dams have already been taken. Geothermal plants are also constrained by geography.
Manufacturing and transportation are hard to replace
2. Fossil fuels are used at every step of renewable energy production. Today, not a single solar panel, wind turbine, hydroelectric dam or nuclear plant has been built without burning oil, gas and coal. It is theoretically possible to replace every use of fossil fuels, but this implies considerable efficiency losses and a much higher cost.
Transportation
Indeed, we should not have the impression that only the quantity of energy matters: quality is also very important. We are not facing an electricity problem — only a fifth of final energy used globally is in the form of electricity. The hard part is to substitute fossil fuels in their non-electric forms. Oil, in particular, is very difficult to substitute since it is both abundant, dense, flexible, movable by pipelines at little energy cost, and easily storable.
Trucks are particularly difficult to replace. Oil is energy dense, and allows the movement of extremely heavy trucks for a reasonable weight. In particular, oil currently allows for the extraction, transport and maintenance of all the other kinds of energy: mining trucks, construction trucks, transporting parts and cement to construction sites, transporting coal, minerals or solar panels to factories, transporting maintenance crews… The list goes on. If trucks stopped running now, all shipments of gas, parts, products, food, medicine, etc. would stop as well. Look around you: what object hasn’t been transported by a truck at some point?
Unfortunately, replacements are much less competitive, because they require too much space or weight. The book “When Trucks Stop Running” (Alice Friedemann, 2015) explored the matter — tellingly, nearly all of the electric truck companies she studied when the book was written have gone out of business since, despite federal and state subsidies. [Edit: Commenter Robin pointed out that Volvo recently issued Electric trucks, with better specs than I expected, like a 300-400 km range - this makes my estimates go upward here. However, we still don't know how affordable they will be - this depends on the lifetime of the battery and how often it has to be changed (400km range is also about half the preferred range for a regular class 8 truck, and charging time would be between 2.5h and 9.5h long). My worry for trucks are now that they take a long time to scale up and need strong material requirements, rather than technical feasibility] The reason is that “even the best theoretical battery has very low energy density compared to petroleum-based fuels [about 100 times less]. Large, heavy vehicles such as trucks, tractors, and cargo ships require batteries too heavy to be practical in most instances, particularly if they are traveling long distances”. Battery work in small, light vehicles, so electric buses, delivery trucks or garbage trucks are common (small range, stop frequently), but heavy-duty ones are rare.
Alternatives exist, but they have drawbacks. Hydrogen and current biofuels are energy vectors: they need energy from another source to be produced. For instance, hydrogen is currently not very competitive to produce using renewables, which is why 96% of it is made from fossil fuels. Even if it were, hydrogen is an energy sink: after all the conversion losses, you can only use about 38% of the energy originally invested. See the hydrogen section. Coal and gas could be made into liquids, but this would only delay things as they are finite too, and would increase greenhouse gasses emissions. Plus, it’s not very efficient, as you lose half the coal’s energy by doing that. See the section on hydrogen.
Biomass (like wood) is the biggest uncertainty I have. It is the current biggest renewable source of energy (with hydroelectricity), at 7%, and depends much less on metals. Moreover, it can be used for trucks, energy and manufacturing (charcoal). Most of the sources I’ve read tend to indicate large limits for scaling up, though. Given current practices, more biomass would compete with agriculture, increase deforestation and consume too much water (see Subramanian et al and Kraxner et al). Most biofuels also have very low energy returns. But, as Dave Denkenbeger points out, there is potential for doing more, especially by using crop and wood residues that wouldn’t compete with food. To what extent needs more research. See the section on biomass.
Manufacturing
The high heat used to smelt metals and cement (currently provided by coal and gas) ahs the same issues as transportation. There are solutions, but they have the same limits, imply a doubling of the price, and changing industrial processes is extremely costly (see the manufacturing section). Finally, oil is used to make many synthetic materials used everywhere: plastics, clothes from synthetic fibers, lubricants, antiseptics, phones, computers, pesticides, asphalt, inks, mosquito nets… Bioplastics can compensate, but it will be slow to scale up and biomass would compete with other uses (see the plastics section).
Then again, it doesn’t mean that it’s impossible to do: those technical challenges are not all necessarily unsolvable. Putting more effort into research could bring up some solutions and improve efficiency. However, the problem arises from the multiplicity and the scale of all the things to replace, and the fact that the solutions will be on average less energy efficient. The book Our Renewable Future, which provides a good overview of the topic (and available for free), addresses some other points, like whether we can replace paints, asphalt and lubricants. It concludes that “In principle, most of the problems we have identified are solvable.[...] But a fully renewable energy future will entail higher costs for building and maintaining infrastructure, and the scale at which manufacturing can take place and infrastructure can be built using only renewable energy is highly uncertain”.
The biggest issue is scale
The most neglected aspects of the energy transition are time and scale. The fossil-fueled global energy system is, sorry for using caps, VERY BIG. I said above that the growth rate of renewables was very strong. However, over the same period, they weren't even able to match the increase in fossil fuels consumption.
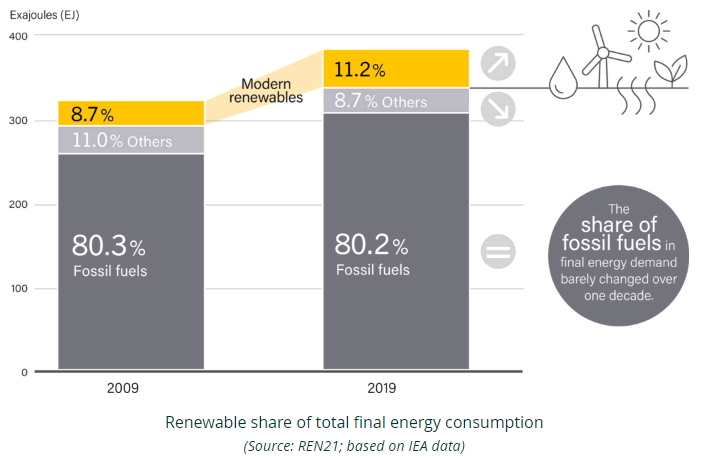
Renewables grew almost 5% per year between 2009 and 2019, outpacing fossil fuels (1.7%). But in absolute terms, the consumption of fossil fuels increased much more than renewable energy.
This means that so far, renewables added up to the global energy consumption instead of replacing them. This means they didn’t have a lot of impact on the climate so far. There has been some progress, since in 2019 renewables accounted for 72% of all new capacity additions worldwide, but we’re still not replacing fossil fuels. Their growth is merely compensating for the decline of nuclear. This brings several insights:
- Energy transitions are long, protracted affairs that unfold over many decades. The inertia of energy systems against changes is large, among others because of the long investment cycles of energy infrastructures or production plants. "In order to counteract path dependence, inertia, and lock-in,[...] one must alter technologies, political and legal regulations, economies of scale and price signals, and social attitudes and values together".
- New technologies are slow to build up: Energy transition expert Vaclav Smil notes that a common mistake is to look at the current growth of renewables, and extrapolate that it will continue to go on forever. Considerable growth is common when starting from a small base - but the real challenge comes later. We often hear about a revolutionary new technology that will save the day, but in practice, “it's taken between 50 and 70 years for any resource to reach a large penetration”. Oil may arguably be the best energy source there is (except for pollution), but even then it took 50 years to capture 10% of the US market, and then 30 more years to reach 25%.
- What is often neglected is the size of the current system: Smil remarks that the world’s fossil-fuel-based energy system, with its infrastructure — coal mines, oil and gas fields, refineries, pipelines, trucks, tankers, filling stations, power plants, transformers— constitutes the costliest and most extensive set of installations and machines that the world has ever built, one that has taken generations and tens of trillions of dollars to put in place. “It is impossible to displace this supersystem in a decade or two—or five, for that matter.”
For nuclear, this is one of the main limits - we’d need to build a 1GW nuclear reactor every two days, for 40 years, to supply global energy. Plus, it needs large upfront investment and thus is unattractive to the private sector; it also depends on specialty metals (among other limits). Fusion faces mostly the same problems, and even with a “fast-track scenario” it could provide only 1% of global energy by 2060. But this is a complicated topic - see the “nuclear power” section.
If fossil fuels go down too quickly, we may not have the energy necessary to build the electric grid, the billions of factories to build solar panels and wind turbines, or the billions of electric cars and trucks. This means that in the meantime, less and less transportation, plastics and manufacturing could be done, and that would increase the price of about every product (including solar panels and wind turbines). This is detailed in full length in the “How fast could an energy transition happen?” section.
What would make me change my mind?
Every week, there is an article about a new energy source or battery that will solve every problem. And 10 years later, most of the time, it still doesn’t exist at any meaningful scale. This is why I learned to be really wary of optimistic articles that in essence say “we’ll just have to do that, problem solved”. There are many alternatives and future technologies that I probably underestimated (I tend to be guilty of that). It’s just hard to separate what works and what doesn’t. So if a new technology is proposed, what I want to know is what are the limits? The book Reality Blind compiled a list of the properties that alternative energy sources should have to be viable. If the alternative fits all of these criteria, I’ll change my mind:
- High net energy return: does it have a high Energy Return on Energy Invested (EROI)? (more about that here). For instance, the energy necessary for space mining or a Dyson sphere would be truly out of this world. The net returns of tidal power or most biofuels are also very low.
- Quality: What can you do with this energy? There is a world of difference between high-density, storable, liquid fuel, and slow-payback intermittent electricity.
- Financial affordability: Can people afford to buy it without going bankrupt? More on investment here.
- Durability: How often do you need to rebuild it?
- Political acceptability: Will people accept it to be deployed next door?
- Speed: How much time will it take to deploy at scale? Will oil start declining before?
- Mature technology: Is this based on commercial technology? Or does it work in the lab but didn’t pass the acid test of real-world deployment?
- Scalability: Electric vehicles, PV solar power, wind, hydro and biowaste all work well on a small scale, especially when supported by a fossil fuel power generation system. But scaling up is the issue.
- Aggregate probability: How many improbable things must go right, on schedule, and what will happen in the meantime?
As you can see, “is it technically possible to do that?” is only a small part of the question.
The real challenge isn’t that no solution exists - we could quote dozens. The issue is that switching to them generally implies a loss in energy efficiency, or takes a higher toll on other natural resources, or are so expensive most people cannot afford them. Otherwise, we would have already made the switch. In the end, that still means less energy globally.
I think a big difference in viewpoint that I have with Dave Denkenberger (ALLFED’s cofounder), one that makes us reach different conclusions, is our relationships to alternative solutions that are currently not commercial, or only exist at a very small scale (<0.1%). Dave is more optimistic as he tends to think that if these solutions have been commercialized, they could be viable with higher prices and will then be deployed at the rate needed. On my side, I am more pessimistic: I tend to think that if something didn’t demonstrate industrial scale feasibility despite decades of research, it means there are very hard challenges lurking in that make wide-scale implementation really difficult. In addition, there are decades for any alternative or new technology to get very big. Counting on them to solve the problem sounds very risky to me. This is a simplification on my part, of course, but given the short delay we have, I simply don’t want to bet my life on that.
My probability estimates
I don’t have a lot of experience at using credences, but I was advised to put mine, so here we go:
- Oil already peaked in 2018: 50%
- Peak oil happens before… 2030: 70% / 2040: 95% / 2050: 98%
- The sum of all fossil fuels will peak before 2050: 93% (the other 7% being that coal scales up in an unforeseen way - bad news for the climate, though)
- After fossil fuels peak, steel, plastics and cement production will decline: 95%
- After fossil fuels peak, metal production will decline: 95%
- Renewables and nuclear cannot scale up fast enough compared to a decline in fossil fuels: 95%
- Energy production will see a decline before… 2050: 90% | 2100: 99% (in the case this isn’t before 2050, this means we would have used much more coal and gas, at the expense of the climate)
Conclusion
I come back to the conclusion of the GTK report, which, from what I found, seems solid:
“A fundamental conclusion is that replacing oil, gas, and coal, using renewable technologies [...] will not be possible for the global human population in just a few decades. There is just not the time, nor resources to do this. What may well happen is a significant reduction of societal demand of all resources of all kinds. This implies a very different social contract and a very different system of governance to what is in place today”.
This doesn’t mean that other energy sources are useless, some can fit a specific role. Biomass could be used as a feedstock to manufacture plastic products, hydrogen should be used for trucks, nuclear power should be used to support manufacturing or heating in the winter. It’s just that matching the scale of fossil fuels is really hard.
I also recommend the article “Energy descent as a post-carbon transition scenario” that provides a good overview of the challenges at hand (thanks to Juan Garcia Martinez of ALLFED for the link). “The case for this is sufficiently plausible that in the energy-intensive developed regions of the world, a post-carbon transition should include policy making and planning for what can be called ‘energy descent’. Reduced overall availability of energy services implies economic degrowth, or downsizing of economies in terms of physical production (Sakai, Brockway, Barrett, & Taylor, 2018).”
Of course, you’d be right to point out that prices will rise, there will be more investment, more substitution, more innovation, and that we’ll try to adapt. We’ll address this, and the consequences of an energy descent on humanity and EA causes, in the next post.
Simon Michaux’s whole argument rests on a straw-man - that renewables need a month of storage by the fanciest batteries to get through winter. This multiplies the minerals factors of hundreds to thousands. But “winter” is not the blind-spot Michaux pretends it is. Engineers have a plan.
OVERBUILD RENEWABLES TO REDUCE STORAGE! Renewables are now 1/4 the cost of nuclear (Lazard) – so we can Overbuild them. Build enough with winter as your target benchmark. 2 days storage is usually enough. The rest of the year generates so much excess so cheap you can do so much exciting stuff with Tony Seba calls it “Super-Power”. They say Australia can probably get away with a 200% renewable grid - that’s just for our electricity grid. https://eclipsenow.wordpress.com/overbuild/
For industrial heating and transport, an Australian industrial think-tank worth a THIRD of our Stock Market! They plan to Overbuild 2020’s electricity grid by 5 TIMES to produce all the products they want to sell and export. https://energytransitionsinitiative.org/
Some energy experts don't even think of it as “Overbuild” - they just model how much will do the job. Extra powerlines are often mentioned as if you overbuild across a wide enough geographic area we can use live power most of the time. EG: Some propose building extra solar out at Perth and HVDC across Australia to the Brisbane, Sydney, Melbourne grid so we can pipe solar power live around the curvature of the earth! Then Perth afternoons power Sydney’s “Duck curve” evenings after dark! HVDC can do this. It only loses 3% per 1000 km. Professor Andrew Blakers explains that a good grid will reduce Storage costs - and that both extra storage and HVDC is about 30% of the total cost. It’s like an entry fee you get to buy heaps and heaps of cheap wind and solar - the other 70% of your grid costs. Or, as this author says:
"Next Michaux overstates the requirement for batteries by at least an order of magnitude by ignoring a few things. First, he ignores the massive HVDC interconnects being built around the world that deflate grid storage requirements. HVDC is the new pipeline (and LNG tanker and oil tanker) after all." https://cleantechnica.com/2023/07/04/how-many-things-must-one-analyst-get-wrong-in-order-to-proclaim-a-convenient-decarbonization-minerals-shortage/
Also at the link above - Michaux falls for the false equivalency of thinking each ton of thermal coal energy MUST be replaced by equivalent wind turbines and solar panels. Which is utter rubbish, because at the same time as we see wind and solar doubling every 5 years (yup, going exponential) we also see efforts to “Electrify Everything” starting. When we “Electrify Everything” the system is so much more efficient we can do away with HALF of the initial energy! EG: Install an off-grid solar + grid battery for little EV’s to come up and recharge at, and you not only avoid those EV’s stressing the grid, you prevent oil tankers driving down the highway each week to deliver fuel. 40% of global shipping is fossil fuels. Once we do this Energy Transition, that’s 22,000 huge steel cargo sheeps we can recycle into yet more wind turbines!
USE PLAINER MATERIALS: all sectors of the energy transition are pivoting away from fancy rare earths and scarcer metals. Why? It’s the cost! Consider the plainer options. Solar is silicon (27% of the earth’s crust!) and aluminium (8%). Wind is aluminium and iron ore (5%) and fibreglass (glass fibres and recyclable plastic polymers.) There are solar panels and wind turbines that do not require ANY rare earths - yes - even in the wind turbine generator! https://eclipsenow.wordpress.com/materials/
Aluminium can replace copper in almost all copper's roles - yes even in EV's. There is 1200 TIMES more aluminium than copper. It recycles well. We will never run out. https://www.shapesbyhydro.com/en/material-properties/how-we-can-substitute-aluminium-for-copper-in-the-green-transition/ Also, all the copper ever mined is still on earth (apart from a few satellites.) We can recycle and re-prioritise that.
FOR 2 DAYS STORAGE - USE PHES! Pumped-Hydro Electricity Storage hardly uses any metal for the enormous energy stored. The best PHES sites are 400 to 800 metres. Michaux’s 1000 page “Report” claimed the sites are too limited. But it had no source! Here it is. https://youtu.be/LBw2OVWdWIQ?t=1342 Are you ready? He tells us the WORLD doesn’t have enough pumped hydro sites because of a viability study about PHES in SINGAPORE! Pancake flat Singapore - their highest hill is only 15 metres? Gee - I wonder why THEY had trouble finding enough sites!? This shows how far outside his comfort zone he is working. He’s a geologist - not an energy expert. Then Dave Borlace from “Just Have a Think” critiqued Michaux. https://youtu.be/Kr_JjO9YWOo?si=A_3j8ZMaTA__pVYy He mentioned one of my heroes, Australia’s Professor Andrew Blakers who won the Queen Elizabeth Prize for Engineering (the Nobel prize for engineers). Blakers shows that if we build OFF-RIVER pumped hydro dams (and pump the water in later), and use satellite data to measure all the best off-river sites - the world has over 100 TIMES what we need. Here’s his youtube intro: http://youtu.be/_Lk3elu3zf4?t=986
His global atlas. Just 1% of these would do all our storage with mainly gravity + water. https://re100.eng.anu.edu.au/global/
Ah, but Michaux replied! He said the amount of water that was required was an extra 50% of the annual fresh water we use. The quantity might be true - but the annual bit is sneaky! See - it would be a once off historical fill. We’d be doing it over time. Say we get keen and do it across 25 years . That’s only an extra 2% per year over that period. If we cover the sites in floating solar to reduce evaporation, the top-up rate is only 10% of the water we CURRENTLY throw at cooling thermal stations like coal. We’ll end up saving water! https://theconversation.com/batteries-get-hyped-but-pumped-hydro-provides-the-vast-majority-of-long-term-energy-storage-essential-for-renewable-power-heres-how-it-works-174446 This is the sort of thing Michaux just omits. It’s not his field. It IS Professor Blaker’s field!
SODIUM GRID BATTERIES are a thing. They need NO lithium, copper, cobalt, or nickel. And we’re not going to run out of sea salt! They are safer and 30% cheaper than lithium. Even if we DID need a month of storage (we don’t!) - Sodium could supply it a million times over. Michaux’s report came out in August 2021 and he claimed sodium was still in the lab. But extraordinary claims require extraordinary evidence - and the commercrial orders had been placed at least a year before.
https://faradion.co.uk/faradion-receives-first-order-of-sodium-ion-batteries-for-australian-market/
Sodium can even be used in EV’s - but it will have a shorter range than lithium. But sodium grid batteries means we can save all our lithium for EV’s. There are many new chemistry’s coming out, but at the moment EV’s are going LFP - Lithium Iron Phosphate. The USGS says there is 89 MILLION tons of lithium. At 6 kg per EV that’s 14 BILLION cars - we only 1.4 billion. Also - we keep finding lithium faster than we’re mining it. In Sept 2023 America just discovered the worlds’ single largest lithium reserves.
Michaux’s OWN PAPER shows we have MORE than enough minerals if we just subtract his ridiculous “Fancy batteries that ate the world”. Check “Michaux Sans Batteries”. https://eclipsenow.wordpress.com/michaux
Michaux interviews on alt-right news like Australia’s Sky News. Climate sceptics are using his work to cast doubt on renewables. Michaux is just a former peak oil doomer trying to justify his doomer manifesto, writing outside his field. As a layperson in this area myself - I avoid people even I can find making outrageous claims, and stick to peer-reviewed research like that of Professor Blakers.
Thank you for the answer. This is the kind of argument I am looking for to update my worldview.
This indeed reduces my confidence in what Michaux proposes. I was not aware of that many different flaws. This is a valuable counter to some of the claims in my posts.
I've depriorized my work on the topic a bit given the difficulty to have an impact there - but I'll try to check your links an update my post.
(btw, I think the reason someone downvoted was because your writing style is not in line compared to forum guidelines, as it might come to some as a bit agressive)
Right now, my worries are less about long-term scenarios where society and scientific research are kept stable until 2050 (as climate scenarios are usually doing).
I'm more worried about the short-term limits on oil production in a world where a lot of time is required to build up renewable or nuclear capacity - especially for new areas like electric trucks, or for scaling up metal production. And the issues this would cause on economic growth, investment capacity, societal trust, and complex worldwide supply chains.
What do you think about this topic ?